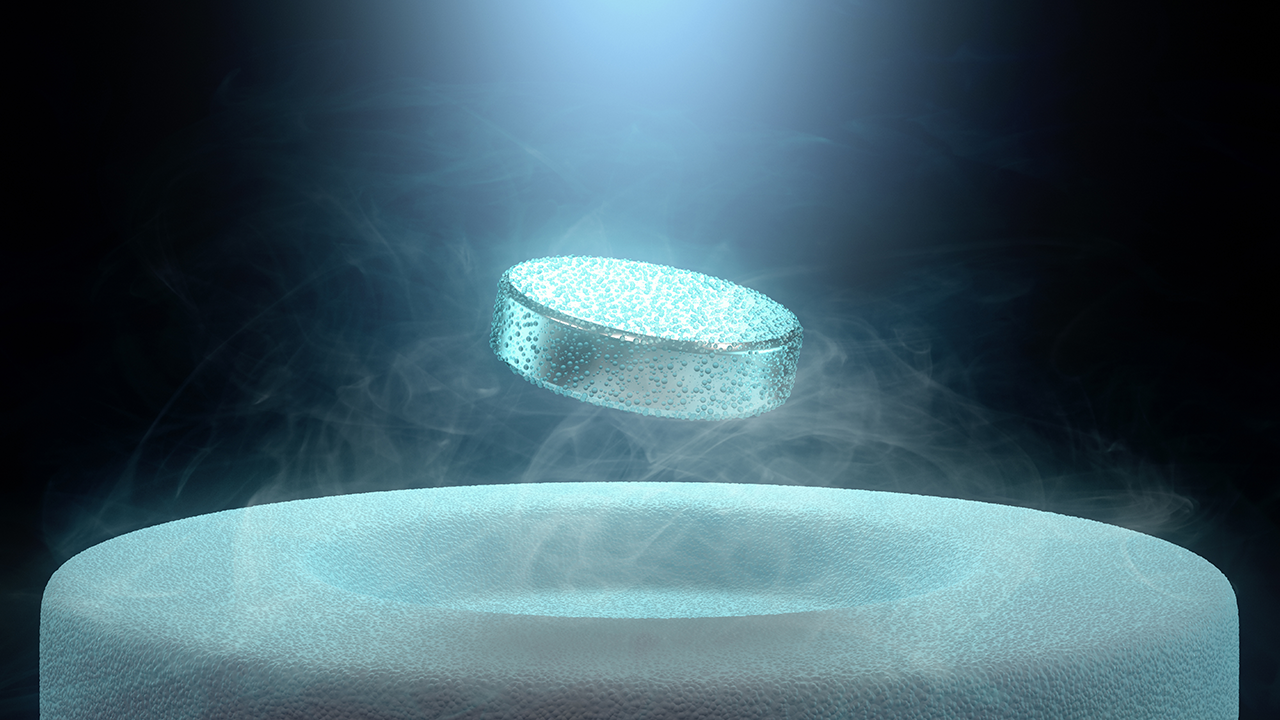
In the physical sciences, superconductors are something of a marvel. Scientists have long known that these materials, under the right conditions, can transfer electricity with zero resistance—losing no electricity in the form of heat. That concept holds promise for a wide range of technological applications, from the electrical power grid to magnetic sensors.
But scientists still grapple with one major question when it comes to ”unconventional” superconductors that exhibit the desirable property of zero resistance already at rather high temperatures: How do they work?
“Superconductors cannot be understood with basic, undergraduate-level electricity magnetism knowledge,” said Martin Greven, Ph.D., a physics professor in the University of Minnesota’s College of Science and Engineering (CSE). “This phenomenon signifies a new quantum state of matter. We still don’t know why some of these materials superconduct.”
Greven is the director of the U’s Center for Quantum Materials, a research team that studies complex oxides, a category of chemical compounds that can be used in superconductors, batteries, data storage, and more that exhibit a wide range of fascinating magnetic and electric properties. The center, established in August 2016 through an initial three-year, $2.6 million grant from the US Department of Energy’s Office of Basic Energy Sciences, aims to discover more about the mechanisms driving these materials’ behavior. The term “quantum materials” refers to materials in which strong interactions among electrons create unusual properties that can so far be observed, but not easily explained.
Along with Greven, the center includes CSE faculty researchers Andrey Chubukov, Ph.D., and Rafael Fernandes, Ph.D., from the School of Physics and Astronomy, along with Bharat Jalan, Ph.D., and Chris Leighton, Ph.D., from the Department of Chemical Engineering and Materials Science, as well as with numerous students and postdoctoral researchers. The synergy between these two academic disciplines is key to the center’s ability to conduct cutting-edge research, Greven said.
The scientists are taking two complementary approaches to explore complex oxides. One is the theoretical approach, which aims to explain why materials behave as they do and adjust our understanding of physics and material sciences to account for it.
“We’re trying to think outside of the box to come up with new concepts, or maybe see if old concepts, tweaked sufficiently, might actually still apply,” Greven said.
The other approach focuses on experimenting with various complex oxide samples, to test and develop compounds that have desirable traits for ultimate use in electronics, electrical engines, the electrical grid, and more. Different chemical arrangements of otherwise similar compounds can yield different properties, so the researchers are creating bulk crystals and thin films out of these chemical compounds, or adding extra oxygen atoms to the materials to manipulate their electric and magnetic properties.
There’s ample room to explore. Between the compounds found in nature and those synthesized in labs, the catalog of known complex oxides is vast—with copper-oxide superconductors alone there are well over a hundred of unique types, Greven said, all made up of stacked sheets of copper and oxygen. The possibilities become even greater when different complex oxides interact. For example, when a film of one complex oxide is covered with another complex oxide, the interface between the two can act completely differently from either of the two compounds individually.
The Path of Zero Resistance
The copper wiring in a typical power cable, despite its relatively low electrical resistance, still loses 7 to 10 percent of the electricity it carries in the form of heat, according to a Nature article. Too high of an electrical current will produce enough heat to melt the wire itself.
With zero resistance, superconductors don’t waste any heat. Wider use of this technology could save tens of billions of dollars globally in energy generation, transmission, and storage losses, according to a report on quantum materials from US Department of Energy. The problem, however, is that many known superconductor materials only work when cooled with liquid helium to extremely low temperatures—in many cases near absolute zero—and that in itself requires energy. In order to superconduct, copper-oxide superconductors only need to be cooled with liquid nitrogen, which is much less costly. Scientists don’t yet understand them sufficiently well to create further improved superconductors that no longer require to be cooled, but these ultimate “high-temperature” superconductors would decrease the financial and energy costs that come with existing superconductors and open them up for new applications, such as in small electronics and electric motors.
A Foundation for Future Technologies
Beyond superconductors, the center’s research could help advance many other technologies that hold great potential, but still face developmental barriers to becoming practical for widespread use, such as ferroelectric RAM, a type of data storage that works similar to a flash drive but holds the potential to “write” data faster and with less energy consumption. Another example is the solid-oxide fuel cell, which is a highly efficient way to produce energy with low emissions, but currently needs to be kept at very high temperatures to function.
In much the same way that today’s electronics are based on semiconductor research first done over 50 years ago, Greven believes the basic research conducted into these applications for quantum materials today provides the foundation for more advanced technologies in the future.
As the center’s Department of Energy-funded work continues, he also hopes to find additional sources of funding, such as from industry partners, to expand the number of people and projects involved in the center. This growth could allow the center’s work to expand beyond complex oxides to other forms of quantum materials and to be fully internationally competitive with quantum materials research taking place across the globe.
In the meantime, Greven said it has been exciting to see how the collection of faculty, research staff, and students involved in the Center for Quantum Materials work together and bridge the fields of physics and material sciences.
“We are able to do things collaboratively that we otherwise wouldn’t have the resources for, and we are more agile in pursuing new lines of inquiry,” Greven said. “It’s a whole new dynamic that makes this research more fun, more interactive, and more productive.”